Recent and future large-scale sea level changes
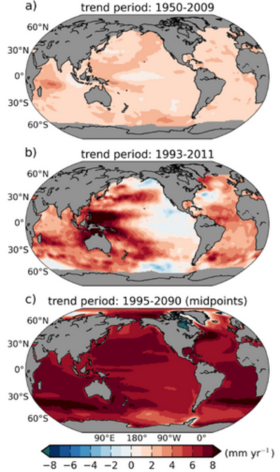
Since the end of the 19th century, global mean sea level is estimated to have risen by about 20 cm, and the rise appears to have accelerated during the past two decades (Rhein et al., 2013). The 2013 IPCC 5th Assessment Report (AR5) projected a global mean rise of ~50 +/-20 cm by 2100 for a medium warming scenario (Church et al., 2013). However, the large thermal inertia of the oceans means sea level will continue to rise during several centuries (IPCC, 2013). While the contribution of glacier and ice sheet mass loss to global sea level change already exceeded the contribution from ocean warming over the last decades (Church et al., 2013), space-based observations revealed that polar ice sheets now lose mass at an accelerated pace (Shepherd et al., 2012). This suggests an even further increase of the cryosphere relative contribution to sea level rise in the future.
Regional contemporary sea level changes on interannual-decadal time scales appear to be mostly steric in nature. Much of the associated anomalies in heat and salt content can be explained by redistributions of the pre-existing water masses, i.e., linked to adiabatic advection associated with changes in ocean circulation (e.g.,Schwarzkopf and Böning, 2011; Fukumori and Wang, 2013). Advective changes naturally involve both thermosteric and halosteric contributions, and their relative role can vary widely depending on the regional temperature-salinity relationship (Köhl and Stammer, 2008; Durack and Wijffels, 2010).
The contribution of climate modes to sea level changes will distinctly differ in the two study regions. More specifically, North Atlantic Oscillation (NAO) impacts are dominant in the North Atlantic and on the European Shelf. In contrast, South-East Asia will be affected by several competing modes, such as El Nino-Southern Oscillation (ENSO), Pacific Decadal Oscillation (PDO), and Southern Annual Mode (SAM). In addition, climate modes are expected to change in the future and thus, quantification of the anthropogenic impact on a changing climate background state and associated oscillations is required.
The main driver of ocean circulation changes on interannual-decadal time scales is wind stress through various dynamic processes (e.g., Ekman pumping, planetary waves, coastal upwelling). In turn, much of this wind-driven variability is related to climate modes, such as ENSO, PDO and NAO. The dominance of such internal redistributions to local sea level changes represents a major challenge for identifying the regional imprints of trends in the net heat and freshwater (and mass) input into the ocean, i.e., the anthropogenic effects responsible for much of the global mean sea level change. Corresponding spatial patterns show large decadal/multidecadal fluctuations, mostly due to internal variability of the climate system (Stammer et al., 2013).
Satellite altimetry has given ample evidence that contemporary sea level changes in many regions of the world ocean are governed by vigorous short-term fluctuations (see Figure), therefore the spatial patterns of sea level trends on interannual-decadal time scales deviate widely from the global mean (e.g.,Meyssignac and Cazenave, 2012). However, because of sparse data coverage by tide gauges, evidence of pre-altimetry regional sea level changes is incomplete at its geographical coverage. Longer-term reconstructions thus need to combine tide gauge records with statistical information on the dominant spatial modes of ocean variability or ocean circulation modeling (with and without data assimilation). Such reconstructions (Fig. a) provide preliminary estimates of evolving past spatial sea level patterns (Köhl and Stammer, 2008; Wenzel and Schröter, 2010) and have enabled to examine the links between coastal erosion and sea level change at sites remote from available tide gauge sites (Yates et al., 2013).
Previous studies suggest that:
(a) global sea level change has been far from uniform even over time periods of 50 years and longer,
(b) the spatial patterns of change differ between interannual and multi-decadal periods, and
(c) the results obtained from different models and methods show non-trivial deviations, indicative of significant remaining uncertainties related to the underlying choices of model configurations and synthesis methodologies.
While anthropogenic effects appear secondary at present-day interannual-to-decadal periods (Fukumori and Wang, 2013), their impact on regional sea level patterns is expected to increase over longer time spans. For instance, possible future trends in the Atlantic Meridional Overturning Circulation are expected to lead to major contributions to the sea level change along the North Atlantic coasts (e.g. Lorbacher et al., 2010).