Resp0nSe
Allocating Responsibility for Regional, Glacier-Related Sea-Level Change (Resp0nSe)
Glacier mass change is one of the main sources of past sea-level rise and glaciers will continue to be a major contributor in the 21th century. Despite their importance, knowledge about past glacier mass change is strongly limited. In the project “Predictability and Attribution of Regional Sea Level Change Caused by Glacier Mass Change (PARSL-Glac)”, funded for the first phase of the Priority Program, we work on attributing glacier mass change to specific radiative forcing, to internal climate variability, and to long-term adjustment of glaciers to past climate change. These findings helped us to quantify the predictability and to reduce the uncertainty of glacier mass change projections. The surprisingly late onset of detectability of anthropogenic glacier mass loss is strongly connected to the long response time scale of glaciers to atmospheric forcing. We quantified the disequilibrium of present-day glaciers with current climate and found that under present day conditions, 36 ± 8 % of the glacier mass found on Earth today is not sustainable and will be lost over the coming centuries. This mass loss is independent of future emissions. Therefore, there is substantial predictability in the glacier contribution to sea level rise.
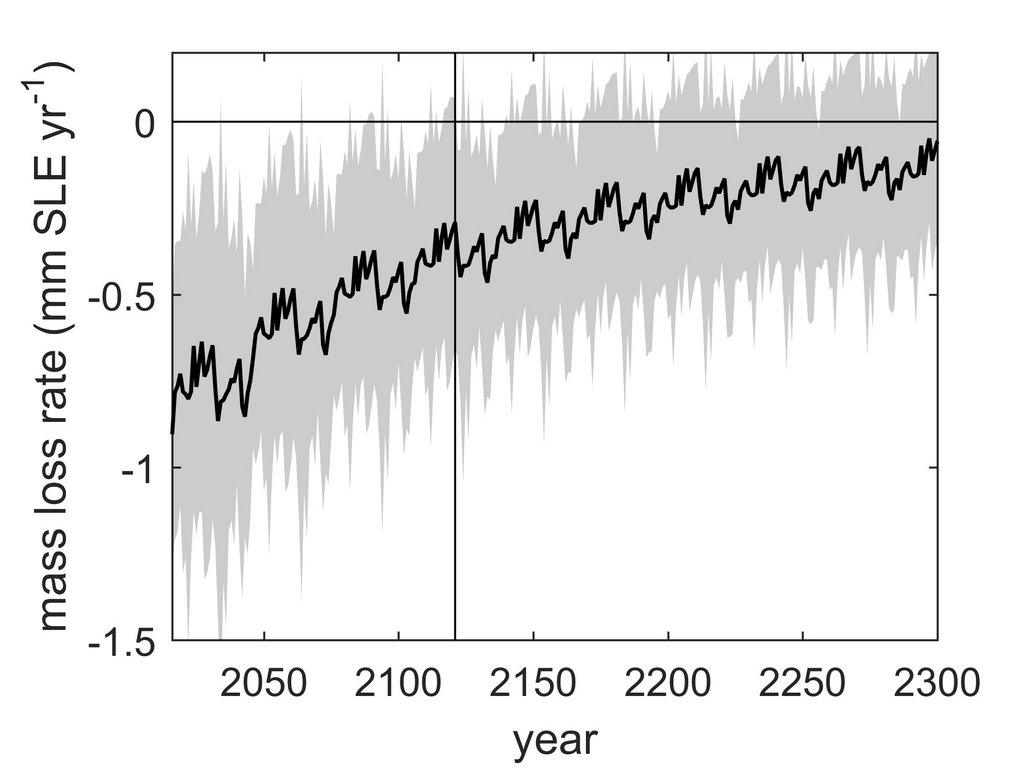
This principle will also help us to reach the goal of this project:
The allocation of responsibility for glacier-related, regional sea-level change to specific past emission pathways.
Specifically, we will:
- quantify the climate sensitivity of the global glacier mass, taking into account its dependence on the background state of the climate system;
- calculate the spatial distribution of this sensitivity, differentiating between different mechanisms of radiative forcing (i.e., CO2 and other long live GHGs, aerosols, and land use change);
- determine the sea-level fingerprints of glacier mass response to radiative forcing of the climate system, again differentiating between different mechanisms;
- combine the information about fingerprints with specific, actual historical emission pathways (e.g., from individual countries) to allocate the responsibility for glacier-related, regional sea-level change to emitters;
- determine the temporal evolution of glacier-related, regional sea-level change caused by a specific emission pathway, most importantly separating between mass change that has been realized, and mass change that is committed for the future;
- validate the approach by applying the global total radiative forcing, to reconstruct the corresponding total global glacier mass loss, and comparing it to reconstructions (i) obtained through direct application of observed and (ii) modelled past climate change, (i) as well as (iii) observations of glaciers.
To give an illustrative example, these steps will allow us to answer questions like the following:
- What is the responsibility of Germany – given its historic emission pathway – for glacier related sea-level change in Indonesia?
- How much of that sea-level change has already been realized, and what did the temporal evolution look like?
- How much of the sea-level change will be realized in the future, and what will the temporal evolution look like?
- What are the uncertainties in this allocation of responsibility?
Our main tool will be the Open Global Glacier Model (OGGM, Maussion et al. 2018, see oggm.org). OGGM is the only available open source model applicable to model glaciers on the global scale. It includes realistic glacier geometry and explicit ice dynamics (i.e., it produces annually updated maps of ice thickness distribution), and a well-established mass balance model applied at regional to global scales (e.g. Marzeion et al. 2012, 2014a, 2014b, 2018). Figure 2 shows an example workflow of OGGM applied to Tasman Glacier, New Zealand.
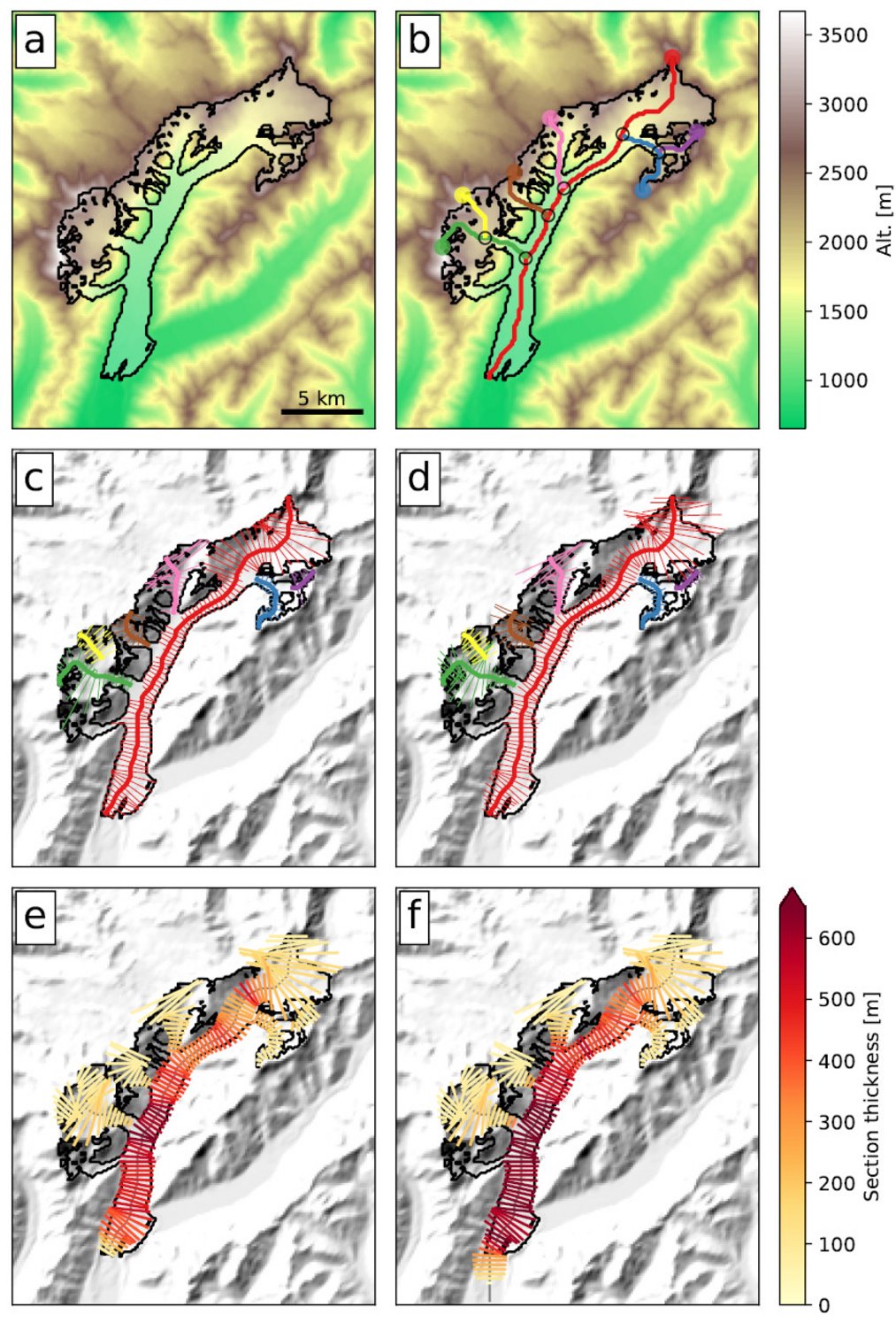
References:
Maussion, F., A. Butenko, N. Champollion, M. Dusch, J. Eis, K. Fourteau, P. Gregor, A. H.Jarosch, J. Landmann, F. Oesterle, B. Recinos, T. Rothenpieler, A. Vlug, C. T. Wild, and B. Marzeion (2019). „The Open Global Glacier Model (OGGM) v1.1“. In: Geoscientific Model Development 12.3, pp. 909–931.DOI:10.5194/gmd-12-909-2019.